Student Research
Key Players of Gene Positioning
Uncovering the noncanonical role of a nuclear export protein in gene targeting
The nuclear pore complex (NPC) interacts with the genome to regulate genome architecture and integrity as well as gene expression. The NPC is composed of ~30 different nuclear pore proteins or nucleoporins (Nups), which physically associate with hundreds to thousands of sites in the genome to modulate transcription in budding yeast, flies, and mammals. In yeast, these Nups can target activated inducible genes to the nuclear periphery, where they experience an increase in transcription. This peripheral localization is not dependent on transcription; however, it requires various players such as transcription factors (TFs), TF-binding sites known as DNA zip codes, Nups like Nup2, and—from our recent findings—exportin-1 (XPO1 or Crm1 in yeast). Using a previously found mutation in a TF that blocks peripheral targeting of various genes, we performed quantitative proteomics and found that the mutation reduced interactions between the TF and Crm1/XPO1. Crm1 is a large essential nuclear export receptor whose known function is shuttling large cargo proteins and RNAs out of the nucleus and into the cytoplasm. When probing the localization of Crm1 in vivo, we not only found a high correlation between Crm1 and Nup binding sites genome-wide and at the enhancers of highly active genes, but also a requirement for Crm1 to mediate interactions between Nups and the genome. Inhibition or loss of Crm1 or Nup2, respectively, causes a major decrease in nascent transcription globally. My work suggests that Crm1 docks TF-bound enhancers at the NPC to enhance transcription. For more details, see: Exportin-1 functions as an adaptor for transcription factor-mediated docking of chromatin at the nuclear pore complex - Tiffany Ge
Unlocking the T-box
RNA form meets function
T-box riboswitches are specialized RNA elements that regulate genes involved in amino acid metabolism in Gram-positive bacteria. They do so by sensing the aminoacylation state of tRNAs. Unlike other riboswitches that respond to small molecules, T-boxes are uniquely equipped to recognize and bind structured RNA with high specificity, making them a key model for studying RNA-RNA interactions. Their role in regulating essential metabolic pathways also positions them as promising targets for novel antibiotics.
To deepen our understanding of this regulatory mechanism, we investigated the translational regulation mediated by the Mycobacterium tuberculosis (Mtb) IleS T-box riboswitch. Our study revealed that both translational and transcriptional T-boxes share a two-step tRNA binding model: first, the tRNA anticodon is recognized to form a partially bound state, followed by the stable binding of the tRNA 3'-NCCA end. Interestingly, the Mtb IleS T-box exhibits longer-lived partially bound states and greater conformational flexibility compared to transcriptional T-boxes, suggesting its adaptation for translation regulation. We also identified two key structural elements—a pseudoknot in the Stem IIA/B-linker and a conserved RAG sequence—that play critical roles in enhancing the stability of the tRNA-bound state through allosteric interactions and conformational constraints.
These findings offer new insights into the mechanistic differences between transcription- and translation-regulating T-boxes, advancing our understanding of RNA dynamics and their potential as targets for disrupting bacterial gene expression. For further details, see Campos-Chavez et al., Translational T-box riboswitches bind tRNA by modulating conformational flexibility. – Eduardo Campos-Chavez
Mediating Transcription
Visualizing human transcription initiation using cryo-electron microscopy
Even though all cells in a multicellular organism contain a copy of the same genome, each cell type requires a unique subset of proteins to carry out its function. The primary regulatory step in protein production is transcribing a gene into RNA. In eukaryotes, transcription of protein-coding genes requires the assembly of a multi-subunit preinitiation complex (PIC) comprised of RNA polymerase II (Pol II), the co-activator Mediator, and a group of general transcription factors. Mediator facilitates the assembly of this complex at gene promoters by engaging both DNA-bound transcription factors and the PIC and regulates transcription initiation by phosphorylating the long, flexible C-terminal domain (CTD) of Pol II. We used cryo-electron microscopy to visualize the structure of the human Mediator-bound PIC. The Mediator head module interacts with Pol II and the middle module serves a structural role. Both the head and middle modules closely resemble the structures of their yeast counterparts, highlighting how conserved this process is across eukaryotes. The less conserved tail module, which serves as a hub for the binding of transcription factors, is much larger than its yeast counterpart. Transcription factor binding sites within the tail module are flexibly tethered to the rest of the complex, which allows for efficient assembly of the PIC across the genome. The structure shows how Mediator positions the CTD of Pol II to be phosphorylated by the kinase CDK7, a crucial step for the processing of the newly synthesized RNA into a mature messenger RNA. For further details, see Abdella and Talyzina et al., Structure of the human Mediator-bound transcription preinitiation complex. – Ryan Abdella & Anna Talyzina
A Balance of Forces
How various motors cooperate to establish and maintain spindle bipolarity in oocyte meiosis
Meiosis is a specialized form of cell division that produces the genetically unique gametes required for sexual reproduction, such as egg and sperm cells. Prior to division, the duplicated chromosomes are separated by rope-like protein polymers called microtubules. In most cells, structures called centrosomes organize these fibers into a spindle shape that emanates from two ‘poles’ on opposite ends of the cell: the microtubules then attach to the chromosomes and pull them apart. Despite not having centrosomes, egg cells, or ‘oocytes’, are still able to arrange their microtubules into a similar bipolar shape. In human oocytes, these ‘acentrosomal’ spindles are quite unstable during meiosis; how these meiotic spindles are formed and maintained in the absence of centrosomes remains poorly understood.
A stabilized spindle requires both microtubules and motors that act upon those fibers to organize large bundles and maintain a bipolar structure. In my work, we developed a strategy that can rapidly remove the motor protein dynein from oocytes of the roundworm Caenorhabditis elegans to better understand how dynein’s activity leads to focused, stable acentrosomal poles at either end of the spindle. These experiments showed that dynein is required both to assemble and stabilize acentrosomal spindles in C. elegans. When dynein and an additional motor protein, KLP-18, were both removed from oocytes simultaneously, the poles blew apart, completely disrupting spindle organization. Surprisingly, I found that the spindles were able to reform and separate the chromosomes. Further probing revealed, for the first time, that a third motor protein (called BMK-1) also helps to organize the spindle into its bipolar structure.
My findings reveal the important role motor proteins play in stabilizing spindles and separating chromosomes in oocytes. As aforementioned, meiosis in human oocytes is prone to mistakes, and these errors are a major cause of miscarriages and birth defects in humans. Therefore, understanding the underlying mechanisms of how oocyte spindles form and remain stable could shed light on why chromosomes sometimes fail to segregate; this may eventually lead to new strategies for combating infertility. For further details, see Cavin-Meza et al., Multiple motors cooperate to establish and maintain acentrosomal spindle bipolarity in C. elegans oocyte meiosis. – Gabriel Cavin-Meza
Geometric Tissue Patterning
Patterning of the Drosophila compound eye via coordinated multicellular morphogenesis
The self-organization of physical form is seen across all spatial scales and stages of biological development, from subcellular patterns occurring in the earliest stages of embryonic development to animal coat patterns that we can see with our eyes. These patterns are predominantly understood to form via "molecular prepatterns," whereby a pattern of cell-cell signaling and/or gene expression is first established in space and then subsequently drives cell differentiation and morphogenesis. Therefore, to understand the self-organization of physical form, one need only to understand how this molecular prepattern is organized.
One system that has been historically characterized to pattern via a molecular pre-pattern is the Drosophila compound eye. The eye is composed of a hexagonal lattice of individual units called ommatidia that are patterned in a wave-like manner. Patterning is believed to be the result of previous columns of ommatidia templating the organization of each new row of ommatidia via diffusible activators and inhibitors. However, the theory underlying the emergence of periodic patterns from such a signaling system doesn’t allow for significant cell movement or shape change (i.e. morphogenesis), which is an assumption we called into question.
To address the contribution of morphogenesis to early eye patterning, we developed two new pieces of technology: (1) methodology to live-image developing eye tissue ex vivo, enabling us to visualize its development in real time using fluorescence confocal microscopy and (2) computational techniques to detect and track thousands of individual cells over the course of 10+ hours of eye development. This quantitative and dynamic data revealed that the wavefront of patterning contained periodic patterns of cellular flow that moved cells into the proper location to differentiate and form a hexagonal lattice. This degree of morphogenetic remodeling, central to the emergence of the final lattice pattern, calls into question a decades-old paradigm of compound eye patterning. For further details, see Gallagher et al., Emergence of a geometric pattern of cell fates from tissue-scale mechanics in the Drosophila eye. – Kevin Gallagher
Metabolizing Methane
Visualizing the active form of a methane-oxidizing metalloenzyme
Particulate methane monooxygenase (pMMO) is the main enzyme used by methane-metabolizing bacteria, methanotrophs, to oxidize and consume methane. Obtaining active pMMO has been challenging since it is a membrane-bound enzyme dependent on copper for activity and loses enzymatic activity through multiple rounds of purification. In this study, we used nanodiscs to recover methane oxidation activity of pMMO by embedding it in a lipid bilayer composed of lipids extracted from the methanotroph membrane. Using single-particle cryo-electron microscopy, we investigated the structural basis of this recovery, revealing a lipid bilayer in the inner pore of the enzyme and stabilized lipids throughout. Critically, we were able to visualize a strictly conserved region of the enzyme for the first time and uncovered a novel copper binding site in this region. This structure gives us a more complete picture of the active enzyme and establishes a new paradigm for investigating the biological oxidation of methane by copper. For further details, see Koo et al., Recovery of particulate methane monooxygenase structure and activity in a lipid bilayer. – Christopher Koo
3'Primed for Differentiation
Epidermal differentiation is promoted by selection of alternative polyadenylation (APA) sites
Epithelial tissue serves as critical barriers for the organs and cavities throughout the human body. This tissue must undergo continuous turnover to compensate for the wear and tear. To regenerate new epithelial tissue, the progenitors need to balance between proliferation and terminal differentiation. To study this homeostasis, we leverage human skin as an accessible research platform. Using this system, we performed 3’READS+ sequencing to characterize for the first time how alternative polyadenylation (APA) regulates epidermal differentiation. This work revealed that epidermal progenitors use intronic poly-adenylation sites to prevent full-length transcription of key differentiation genes such as GRHL3. We found that this was mediated through the Cleavage and Polyadenylation Specificity Factor Complex (CPSF) along with the RNA-binding protein HNRNPA3. Overall, this work highlighted a novel role for APA in regulating epidermal differentiation and emphasized the importance and prevalence of post-initiation control over transcription. For further details, see Chen, Lloyd, et al., Epidermal progenitors suppress GRHL3-mediated differentiation through intronic polyadenylation promoted by CPSF-HNRNPA3 collaboration. – Sarah Lloyd
Nano-environmental Engineering
Measuring, modeling, & modulating the chromatin nano-environment for transcriptional regulation
Advances in molecular biology have made it easier than ever to explore the functions of the human genome. We tend to now think of the functional properties of the genome with respect to the activities each gene carries out and the overarching molecular regulators of that behavior. Comparatively underexplored is the physical organization of the genome and its effects on the functions of genes. In much the same way that the organization of a city influences our behavior (e.g. driving in Boston vs. Chicago, public transit in Los Angeles vs. NYC), the structure of the genome has a major impact on molecular behavior. To study the effects of physical organization on genomic function requires the capability to measure the relevant structures in real-time, develop models of how this organization alters behavior, and test them experimentally. My research has therefore focused on all of these aspects – helping to develop microscopic techniques that are nanoscale sensitive to image the physical structure of the genome within seconds, developing mathematical models that account for the role of the measured physical structure on processes such as gene expression, and testing these predictions using molecular techniques.
While the understanding of the role physical forces play on genomic function is at its earliest stages, we have been able to apply this approach to develop compounds that control the physical structure of the genome in order to manipulate global patterns in gene expression, so called “Macrogenomic Engineering.” My goal is to develop this physico-chemical Macrogenomic Engineering as a complement to existing gene editing tools: while gene editing techniques work at the level of the linear genetic code and thus individual genes, the regulation of the genomic structure affects the global patterns of gene expression. As most diseases are inherently genomic and multifactorial in nature, the combination of these techniques may eventually allow us to address diseases where the global reprogramming of gene expression plays a role, such as cancer, inflammation, and neurodegeneration. For further details, see Almassalha et al., The Global Relationship between Chromatin Physical Topology, Fractal Structure, and Gene Expression. – Luay Almassalha
Forming Brain Tissue
Uncovering how integrin is needed for cells to come together and assemble organs in regeneration
The use of stem cells to regenerate damaged tissue has the potential for far-reaching medical applications. Certain animals have evolved the ability to harness their stem cells for complete regeneration after injury and are models for understanding natural mechanisms of tissue repair. The flatworm planarian Schmidtea mediterranea is famous for its ability to regenerate after removal of any body part because of robust mechanisms to control the activity of its potent adult stem cells. My research uses planarians as a model system to study how stem cells differentiate into specialized cell types and how their progeny assemble to form functional organs, such as the brain, during regeneration. We found that β1-Integrin, a cell surface molecule involved in adhesion to the extracellular matrix, is required for the proper organization of brain tissue in planarian regeneration. Inhibition of the β1-Integrin gene caused regenerating planarians to generate disorganized brains in which neurons aggregated with each other randomly rather than forming distinct and symmetric brain lobes. Intriguingly, these aggregates resemble organoids, a type of synthetic tissue that human stem cells form in petri dishes in the absence of a natural environment for growth. Planarians depleted of integrin activity also produced excess brain neurons that were placed at abnormal locations, suggesting that integrin signaling is additionally required to determine appropriate numbers and positions of mature brain neurons formed through regeneration. Together these results showed that integrin signalling regulates planarian stem cell activity to control the organization and scale of tissues formed during regeneration. For further details, see Bonar & Petersen, Integrin suppresses neurogenesis and regulates brain tissue assembly in planarian regeneration. – Nicolle Bonar
Genomics in C. elegans
Leveraging genomic and computational approaches to understand biology
I have a long-standing interest in the application of novel computational tools to answer important biological questions. During my PhD studies in the IBiS program at Northwestern, I focused on understanding the genomes of a tiny translucent nematode called Caenorhabditis elegans. Specifically, I studied the role genetic differences play in making each individual in a population distinct from one another. My projects have focused on three areas. First, I characterized natural differences in the genomes of wild strains by developing bioinformatic pipelines to identify single nucleotide variants (SNVs) and other genomic features. Second, our lab measures correlations of these genetic differences with physical differences (using a technique known as genome-wide association) to identify which specific genetic differences make individuals distinct from one another. For example, we identified a specific genetic difference that contributes to the regulation of the ends of chromosomes (telomeres) across the natural population. Finally, I developed tools that make the study of natural variation across the species easier for the scientific community. Towards this end, I created an interactive web portal - The C. elegans Natural Diversity Resource - available at elegansvariation.org, and developed a command-line utility for processing genome sequence data known as VCF-kit. For further details, see Cook et al., CeNDR, the Caenorhabditis elegans natural diversity resource. – Daniel Cook
Shapeshifting Proteins
How intrinsic motions help nuclear receptors bind ligands and relay signals
In solution, proteins are dynamic, flexing or shifting between different shapes (aka conformations). These motions are known to have profound effects on protein function. My work focuses on studying the intrinsic motions (as opposed to motions caused by ATP-binding or -hydrolysis) of an important family of transcription factors known as nuclear receptors. These proteins are found throughout the animal kingdom and regulate fundamental biological processes such as metabolism, growth, development, and homeostasis. Nuclear receptors bind to small molecules that serve as signals from different parts of the organism as well as the external environment. This makes them effective targets for small molecule drug therapies; understanding how they sense and respond to these signals is an active area of research. It is well known that nuclear receptors undergo conformational changes upon binding to small molecule ligands, switching the receptor between active and inactive states. The presence of these motions and their functional implications in so-called orphan or ligand-independent receptors has not been explored in solution at physiologically relevant temperatures. I am using nuclear magnetic resonance (NMR) spectroscopy (which is related to MRI in medicine but is a useful tool for studies of molecules), along with a variety of other biophysical, biochemical, and computational techniques, to characterize these motions occurring in the ligand-binding domains of several orphan nuclear receptors in solution. My studies of the Drosophila NR5A receptor, Ftz-F1, have shown that the static picture implied by x-ray crystallographic analysis might be overly simplistic and that a short helix in the ligand-binding pocket is dynamic, undergoing motions on multiple time- and length-scales with implications in binding to small molecules and coregulators. For further details, see Daffern et al., Solution Nuclear Magnetic Resonance Studies of the Ligand-Binding Domain of an Orphan Nuclear Receptor Reveal a Dynamic Helix in the Ligand-Binding Pocket. – Nicolas Daffern
A Needle in a Haystack
Magnifying naturally rare replication errors in DNA
DNA polymerases are nature’s photocopiers. They are able to replicate DNA with astonishing accuracy and ensure faithful propagation of our genomes. Although rare, replication errors accumulate over time and in turn drive critical biological phenomena such as evolution and disease. The mechanisms which govern DNA polymerase error propensity are modulated by a myriad of environmental factors, ranging from the divalent metal cation used for catalysis to the sequence context of the DNA being copied. Advances in next-generation sequencing have made it easier to begin mapping the impact of such conditions on DNA polymerase fidelity. However, because most DNA polymerases rarely make mistakes, it can be challenging and resource-intensive for even the most sophisticated technologies to detect such infrequent errors. To overcome these obstacles, I have developed a technique that amplifies the naturally low error rates of DNA polymerases in order to paint a high-resolution picture of DNA polymerase error preferences. In addition to magnifying errors, this method includes a built-in repository of hundreds of sequence contexts, allowing for the systematic discovery of unique sequence-based modulators of DNA polymerase fidelity. For further details, see de Paz et al., High-resolution mapping of DNA polymerase fidelity using nucleotide imbalances and next-generation sequencing. – Alexandra de Paz
It's about Time!
A superior algorithm to infer gene regulatory networks from time-series expression data
Many genetic components are involved in diseases such as cancer, and genome-scale experiments now allow us to uncover information about them faster than ever. Untangling how genes interact is essential for developing more effective treatments for disease. An emerging approach to this problem is to infer gene regulatory networks (GRNs) from microarray or RNA-seq experiments. GRNs condense thousands of changes in gene expression into a map that depicts the flow of information through genes using directed edges. Although the computational tools for doing this have consistently improved, many do not incorporate temporal information from time-series measurements, leading them to make false predictions.
We developed a novel algorithm that improves network inference from time-series gene expression data, which we call Sliding Window Inference for Network Generation (SWING). The key idea behind SWING is to account for time delays between regulatory events that exist due to the many steps required for genes to respond to stimuli. SWING partitions experimental data into many small "windows," which we found improves algorithm accuracy for revealing interactions that occur within short periods of time. It also pairs this with the concept of Granger causality—a statistical idea from economics that states that an event can be forecasted by another causal event in the past—to improve the inference of GRNs from time-series gene expression data. Using simulated GRNs, where we know the true interactions, we show that SWING can reconstruct network maps more accurately by accounting for time delays. We identify many time-delayed interactions from simulated systems and in vitro E. coli and S. cerevisiae data, and show that SWING can improve network inference in these contexts. Overall, we demonstrate the importance and utility of accounting for temporal ordering to understand biological systems. In other words, it’s about time! For further details, see Finkle, Wu, and Bagheri, Windowed Granger causal inference strategy improves discovery of gene regulatory networks. – Justin Finkle & Jia Wu
Understanding Stem Cells
Exploring how cell-cell communication mediates cell fate decisions in the early embryo
Stem cells of the early embryo, termed embryonic stem cells, will give rise to all the cell types of the mature organism. Cells are eventually partitioned into one of three different tissue layers, called germ layers, with each preparing cells for a very different function. Neural crest stem cells, which are unique to vertebrate organisms, are part of a germ layer where cells are destined to become either skin cells or neural cells, yet they are not instructed to become either of these specialized cell types. Instead, these cells exhibit a stem cell-like state until later stages of development to make contributions to mature structures like muscle, cartilage and bone, and the valves of the heart. These cells resist the push to become specialized and provide a great system to answer the enigmatic question, how do stem cells maintain their remarkable potential? My work uses Xenopus as a model organism to explore how cell-cell communication is involved in regulating stem cell potential, providing novel insights into the cell signals that are essential for both stem cell pluripotency and for neural crest formation. I found that fibroblast growth factor (FGF) signals regulate the gene expression of pluripotent stem cells, and that there is a striking switch in the signaling cascades activated by FGF signals as cells exit the stem cell state and commence lineage restriction. Specifically, pluripotent stem cells display and require Map Kinase signaling, whereas PI3 Kinase/Akt signals increase as cells undergo lineage restriction. Importantly, retaining a high Map Kinase/low Akt signaling profile is essential for establishing Neural Crest stem cells. Thus, my work sheds important light on the signal-mediated control of pluripotency and the molecular mechanisms governing the genesis of the Neural Crest. For further details, see Geary & LaBonne, FGF mediated MAPK and PI3K/Akt Signals make distinct contributions to pluripotency and the establishment of Neural Crest. – Lauren Geary
What Are Methanobactins?
The biosynthesis, regulation, and transport of an unusual family of metal-binding natural products
Methanotrophic bacteria – bacteria for which the only source of carbon is methane – are a major area of research for the Rosenzweig group. The enzymes responsible for the oxidation of methane to methanol are metalloenzymes, and metal homeostasis plays a major role in governing methane oxidation. My project focuses on the natural product family known as methanobactins. These compounds are secreted from the cells of methanotrophic bacteria, and once outside of the cell, they bind copper with high affinity. Copper-bound methanobactins are then re-admitted to the cell and the internalized copper is ultimately incorporated into some of the metalloenzymes responsible for methane oxidation. Over my time in the Rosenzweig group, my work has provided insight into how these unusual natural products are biosynthesized, how they are transported out and into the cell, and how their production is regulated by the availability of copper. For further details, see Kenney et al., The biosynthesis of methanobactin. – Grace Kenney
Positioning Organelles
Uncovering a role for mitochondrial anchors in nuclear migration
Inter-organelle contacts facilitate communication between organelles and impact fundamental cellular functions. Investigations into the molecular mechanisms of inter-organelle tethering are still in the early stages, and we are just beginning to appreciate the number and variety of inter-organelle tethers that exist. My work is focused on understanding how cells establish, maintain, and alter contacts between organelles at the right time and place to impact a wide array of cellular functions. I am studying an organelle contact site in the model organism budding yeast. Using live-cell microscopy to visualize organelles and contact site proteins, I found that the assembly of a mitochondria-ER-cortex anchor, called MECA, requires mitochondria. Once assembled, MECA persistently anchors mitochondria to the cell cortex. MECA also serves as a cortical anchor for dynein during mitotic spindle positioning. I found that disrupting the mitochondria-dependent assembly of MECA leads to defects in dynein-mediated spindle positioning. Therefore, MECA-mediated mitochondria-plasma membrane tethering not only impacts the spatial distribution of mitochondria within cells but also the position of the spindle. This work helps us to understand how the regulated assembly of a contact site integrates the positioning and inheritance of two essential organelles and expands our knowledge of organelle contact site function. For further details, see Kraft & Lackner, Mitochondria-driven assembly of a cortical anchor for mitochondria and dynein. – Lauren Kraft
Structures in Motion
Visualizing conformational dynamics underlying activation and modulation of a unique family of GPCRs
A cell’s ability to detect, decode, and respond to external stimuli is essential for maintaining homeostasis. In humans, G protein-coupled receptors (GPCRs) are the dominant cellular signal processors and form the largest family of membrane receptors. Due to their involvement in nearly all physiological processes, GPCRs have become the largest family of drug targets – currently targeted by ~40% of FDA approved pharmaceuticals. Therefore, understanding how ligands affect GPCR structure to regulate signaling is a central question in both biology and medicine.
My research in the Vafabakhsh Lab focuses on understanding the dynamic activation and modulatory mechanisms of a unique family of GPCRs, the metabotropic glutamate receptors (mGluRs). mGluRs are predominately located in synapses of the brain and are promising therapeutic targets for a range of neurological and psychiatric disorders. mGluRs are unique as they function as constitutive dimers and possess large extracellular domains that separate the ligand-binding and signaling sites. Basically, ligand binding (glutamate) causes a local conformational change that is then propagated 120Å and across three distinct structural domains to reach the inside of the cell where it activates G protein pathways. To visualize this process, I used single-molecule FRET (smFRET), essentially a nanoscale ruler, which allowed me to measure receptor motion with high sensitivity and in real-time! smFRET requires the attachment of two fluorophores to the receptor, without disturbing its structure. To achieve this, I used engineered translational machinery that allowed site-specific incorporation of unnatural amino acids that contained chemical groups for fluorophore attachment. Using our novel conformational sensor, we found that mGluR2 constantly transitions among four states, two of which were previously unknown, and that transitions between the states occur in a sequential manner. In addition, we showed that the newly identified intermediate states act as critical checkpoints for activation and that mutations can alter their stability, in turn affecting receptor signaling – posing a novel approach for modulating receptor function. For further details, see Liauw et al., Conformational rearrangement during activation of a metabotropic glutamate receptor. – Brandon Liauw
Cutting in on Trafficking
The assassin DrICE is required for a process that doesn’t end in death: intracellular trafficking.
Perhaps counterintuitively, cell death is an essential part of life – from sponges to humans, almost all organisms experience some form of programmed death (apoptosis) that is carried out by genetically encoded proteins. In apoptosis, a death stimulus triggers the activation of the executioners of cell death called caspases. Although caspases are infamous for their ability to cause cell death, it has recently been discovered that these deadly proteins are also required for essential non-apoptotic functions such as stem cell differentiation and sperm cell maturation.
In our work, we have discovered that the caspase DrICE is required for cell growth; we demonstrate that DrICE is both necessary and sufficient for the proper elongation of the tube-shaped Drosophila tracheal system (airway). DrICE affects tracheal growth by altering the intracellular trafficking of cargo, including signaling molecules and components of cell-cell junctions. Together with other newly discovered non-apoptotic caspase functions, our results raise many critical questions about the roles of caspases in trafficking: What are the proteolytic targets of endocytic-associated caspases? How can caspase activation be limited to regulating endocytosis without triggering full-on cell death? What came first in evolution – apoptosis or non-apoptotic caspase functions? With answers to these questions, we can begin to better understand how alterations in intracellular trafficking might occur and contribute to disease progression in various contexts including cancer, in which cancerous cells have chronically activated caspases. For further details, see McSharry & Beitel, The Caspase-3 homolog DrICE regulates endocytic trafficking during Drosophila tracheal morphogenesis. – Saoirse McSharry
Bacterial Methane Consumption
Elucidation of the copper cofactors responsible for driving enzymatic methane oxidation in vivo
Methane is an abundant, highly-potent greenhouse gas, and methane-oxidizing bacteria (MOB) are the primary natural methane sink. As such, MOB are instrumental in mitigating methane emissions, both anthropogenic and natural. The predominant catalyst of aerobic methane oxidation in MOB is the enzyme particulate methane monooxygenase (pMMO), which oxidizes methane to methanol, thereby breaking the strongest C-H bond of any alkane. The mechanism by which pMMO catalyzes this chemistry, particularly the nature of the endogenous metallocofactors that drive reactivity, have confounded researchers for decades. My work in the Rosenzweig and Hoffman labs has centered around solving this problem, predominantly through biochemical and spectroscopic tools. I was able to define the pMMO metallocofactors as two monocopper sites, thereby providing a roadmap for future studies of pMMO reactivity. Specifically, by taking advantage of the limited number of paramagnets present in the MOB, I was able to combine in vitro and in vivo paramagnetic resonance spectroscopic results to elucidate the structure of the two monocopper cofactor sites. This finding is especially remarkable considering a monocopper site capable of methane oxidation under physiological conditions is unprecedented. For further details, see Ross et al., Particulate methane monooxygenase contains only mononuclear copper centers. – Matthew Ross
The Vitruvian Planarian
Controlling the size of tissues during regeneration
Since ancient times, philosophers and scientists have been interested in how animals organize cells to create the relative proportions of tissues. For example, Da Vinci’s famous “Vitruvian Man” depicts the symmetrical proportions of the human body. The establishment of tissue proportions during development is a fundamental process critical for all animal life. For example, the developing heart grows to the proper size needed for the body, and no larger or smaller. Some animals have the ability to reestablish their form after injury, a process called regeneration. The flatworm planarian Schmidtea mediterranea can regenerate from nearly any injury. I utilize planarians as a model system to study the control of tissue scale because of their ability to regenerate tissues in the appropriate proportion relative to pre-existing tissue before injury. In order to better understand tissue size control, we inhibited signaling molecules in planarians and observed changes to the size of body regions of the animal. Inhibition of the gene mob4 caused animals to grow tails of more than twice the normal size. mob4 acts within stem cells to inhibit the production of posterior signaling cells normally restricted to the posterior terminus of the animal. This work suggests that the production of a signaling center from stem cells at the animal terminus is fundamental in controlling the relative size of tissues. For further details, see Schad and Petersen, STRIPAK Limits Stem Cell Differentiation of a WNT Signaling Center to Control Planarian Axis Scaling. – Erik Schad
DNA Passage Caught by CryoEM
Visualizing transient interaction states between gyrase and DNA using cryoEM
Topoisomerases are enzymes present in all domains of life. They are responsible for maintaining the proper winding and linking of DNA molecules during the challenging processes of transcription and replication. Once a DNA double helix becomes unwound and the two strands separated, tension is formed in front and behind the replication or transcription fork. Unless the tension is properly removed by a topoisomerase, the transcription/replication machinery will be unable to move forward and DNA could break, which will have a fatal effect on cells. In addition, after replication, topoisomerases assure that the old and new DNA strands do not get tangled together. Participation in these crucial cellular processes makes topoisomerases perfect targets for antibiotics and chemotherapeutics. In particular, DNA gyrase, a unique enzyme responsible for maintaining the naturally underwound state of DNA in bacteria, is an antibiotic target in illnesses caused by, for example, S. pneumoniae,M. tuberculosis, and B. anthracis. DNA gyrase is a topoisomerase built by two gyrA and two gyrB subunits. Together they bind DNA, introduce a transient break in DNA, and utilize the energy from ATP hydrolysis to change the gyrA dimer conformation from closed to open while separating the broken strands. Once the DNA strand is open, another strand can pass through the break. After passage, the break is resealed and gyrA returns to its closed conformation resulting in underwound DNA. In my research, I was able to trap a DNA strand passing through the cavity of an open gyrA dimer. This state, which has never been observed before, is critical for understanding the protein-DNA interactions in gyrase’s mode of action. For further details see Soczek et al., CryoEM structures of open dimers of gyrase A in complex with DNA illuminate mechanism of strand passage. – Katarzyna Soczek
Memories of Transcription
Mechanism and evolution of GAL gene epigenetic transcriptional memory
How do cells remember previous experiences? One way is through epigenetic processes in which a stimulus can induce heritable (although not necessarily permanent) changes in behavior or phenotype. Transcriptional memory is such a phenomenon: certain inducible genes show a much faster reactivation for several generations after they were previously expressed. This type of memory has been described in budding yeast, fruit flies and humans. For my thesis project, I performed a genetic screen for mutants in budding yeast that were disrupted for memory of previous growth in galactose, which activates the GAL genes. This type of transcriptional memory lasts for eight generations and provides a huge adaptive advantage in galactose, which is a sub-optimal sugar. I found that a point mutation in the co-activator Gal1 specifically blocked memory by disrupting a physical interaction with its target. Because Gal1 is made at high levels when cells are grown in galactose, this supported the idea that proteins produced in the first round of activation can serve to promote faster reactivation for several generations through cytoplasmic inheritance. I also found that Gal1 stimulates an inter-domain interaction within Gal4 transcription factor, leading to stronger expression of GAL genes. By comparing how different Saccharomyces species adapt to galactose, I found that GAL transcriptional memory evolved from an older, constitutive poising mechanism that is genetically encoded through low level, basal expression of GAL1. My work suggests that the evolution of GAL transcriptional memory in budding yeast is the product of competing pressures to retain the fitness benefits of previous growth in galactose while maximizing fitness in glucose-galactose mixtures. For further details see Sood & Brickner, Genetic and Epigenetic Strategies Potentiate Gal4 Activation to Enhance Fitness in Recently Diverged Yeast Species. – Varun Sood
Stochastic Gene Positioning
Eukaryotes organize their genome through stochastic diffusion and nuclear architecture interaction
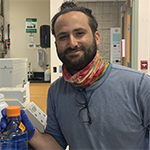
These and other student projects have led to many awards and papers.